14.12.2023
Exploring the Basics of Hydrogen Electrolysis
In the fast-paced world of industrial production, it’s a top priority to find sustainable solutions that meet the demands of modern industry and address environmental concerns. Hydrogen, as a clean energy carrier, has gained significant attention in recent years.
One of the key methods for hydrogen production is hydrogen electrolysis. In this article, we will explore the various methods of hydrogen electrolysis, with a focus on alkaline electrolysis, and its role in transforming industries such as glass and ceramics, concrete production, steelmaking, and fertilisers.
Introduction to Hydrogen Electrolysis
Water electrolysis, sometimes also referred to as hydrogen electrolysis is the process of splitting water (H2O) into its constituent elements, hydrogen (H2) and oxygen (O2), using an electric current. This is done through the use of an electrolyser, a device that contains two electrodes and an electrolyte separating them. When an electric current is applied between the electrodes, water molecules are separated into hydrogen and oxygen gases.
The key advantage of hydrogen as an energy carrier lies in its potential to be produced with renewable energy sources, such as wind, solar, and hydropower. This green hydrogen production holds great promise for reducing the environmental impact of various industries.
The main methods of Hydrogen Electrolysis
There are three prominent methods of hydrogen electrolysis: alkaline electrolysis, proton exchange membrane (PEM) electrolysis, and solid oxide electrolysis. Each method has its unique characteristics and applications. However, the general chemical reaction is the same for each type of electrolyser.
- 2H2O(l) → 2H2(g) + O2(g)
Alkaline Electrolysis
Alkaline electrolysis is one of the most established methods of hydrogen production. Alkaline electrolysis is a process that involves splitting water molecules (H2O) into hydrogen (H2) and oxygen (O2) using an alkaline electrolytic cell. This method uses an alkaline electrolyte solution, typically potassium hydroxide (KOH) or sodium hydroxide (NaOH), which acts as a conducting medium to facilitate the flow of ions between electrodes.
The electrolysis cell consists of two electrodes, an anode, and a cathode, submerged in the alkaline electrolyte solution. The anode is typically made of a durable and corrosion-resistant material such as nickel; the cathode can also be made of nickel, stainless steel, or other metals.
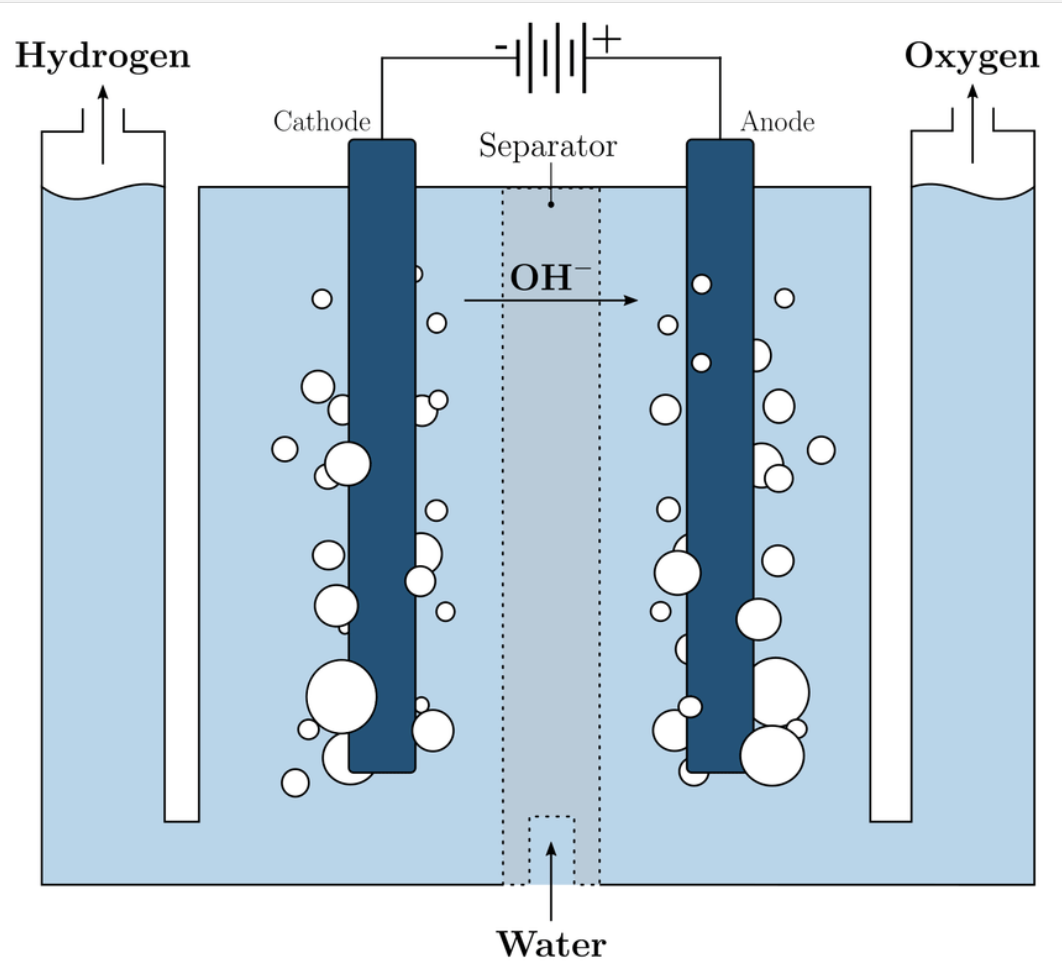
When an electric current is passed through the electrolyte solution via the electrodes, several reactions take place at the anode and cathode:
- At the anode, oxidation of water molecules occurs, leading to the release of oxygen gas (O2).
- The reaction at the anode can be represented as: 4OH- -> O2(g) + H2O(aq) + 4e-
- At the cathode reduction of water molecules occurs, leading to the production of hydrogen gas (H2).
- The reaction at the cathode can be represented as: 2H2O(l) + 2e- → H2(g) + 2OH-(aq)
Overall, the electrolysis of water using alkaline hydrogen electrolysis can be summarized by the balanced equation: 2H2O(l) → 2H2(g) + O2(g)
Proton Exchange Membrane (PEM) Electrolysis
PEM electrolysis, which stands for Proton Exchange Membrane hydrogen electrolysis, is another method used for splitting water into hydrogen and oxygen. The PEM electrolysis cell consists of two electrodes, an anode, and a cathode, separated by a solid polymer electrolyte membrane, typically made of a specialized proton-conducting material like Nafion. The anode and cathode are usually made of precious metals such as platinum, but efforts are ongoing to develop more cost-effective alternatives. When an electric current passes through the PEM electrolysis cell, the following reactions occur at the anode and cathode:
- At the anode, water oxidation takes place, resulting in the release of oxygen gas (O2).
- The reaction at the anode is represented as: 2H2O(l) → O2(g) + 4H+(aq) + 4e-
- Formed hydrogen ions (protons) migrate through the electrolyte to the cathode. At the cathode, reduction of protons occurs, leading to the production of hydrogen gas (H2).
- The reaction at the cathode is represented as: 4H+(aq) + 4e- → 2H2(g)
Overall, the PEM hydrogen electrolysis process can be summarised by the balanced equation:
2H2O(l) → 2H2(g) + O2(g)
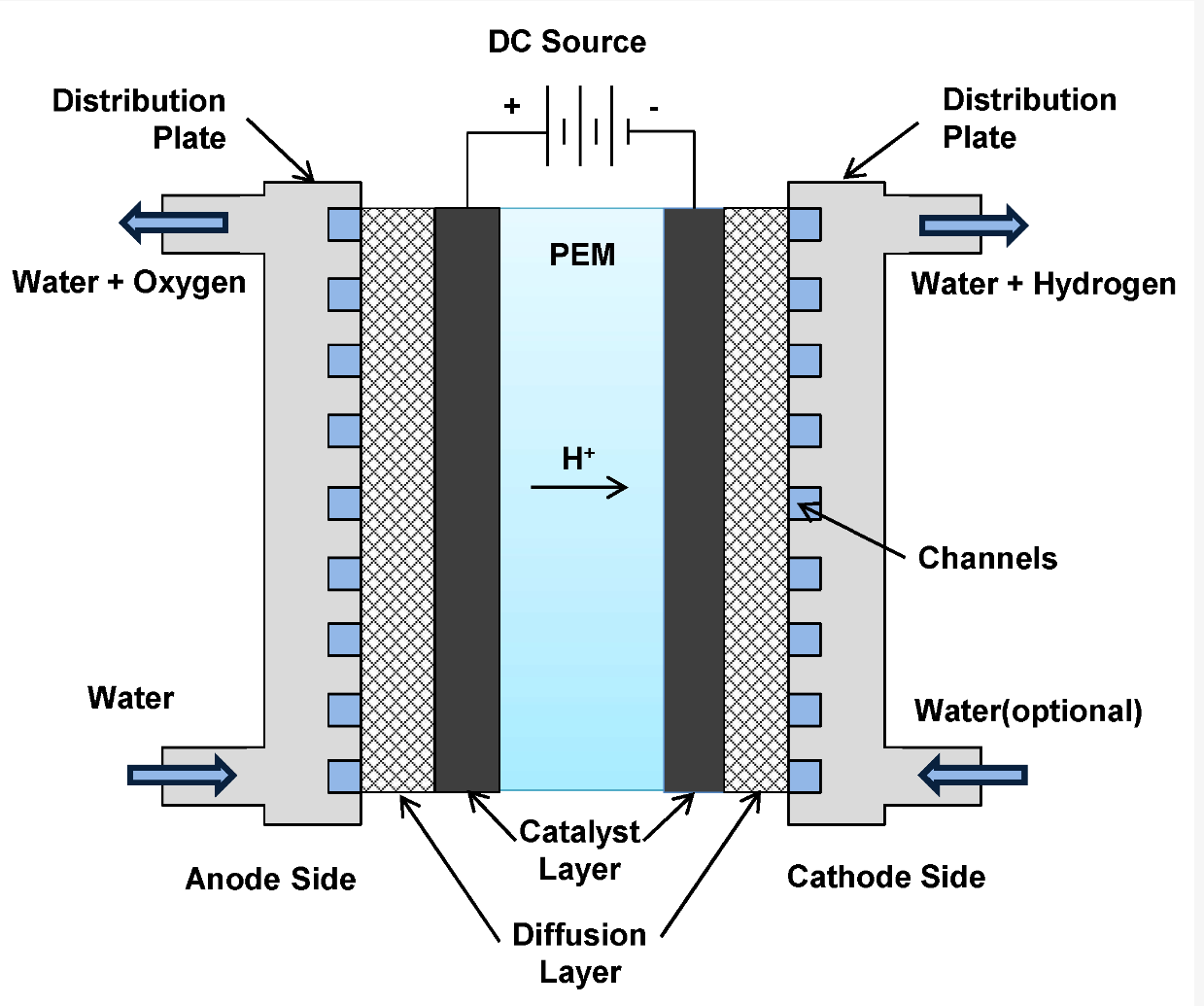
The solid polymer electrolyte membrane used in PEM electrolysis plays a crucial role by allowing the passage of protons (H+ ions) while preventing the mixing of hydrogen and oxygen gases. This design ensures the selective separation of the generated gases and minimizes crossover, improving the efficiency of hydrogen production.
The PEM electrolyser is compact and best suited for small-scale applications.
Solid Oxide Hydrogen Electrolysis
Solid oxide hydrogen electrolysis is a process that uses a solid oxide electrolyte to split water into hydrogen and oxygen gases at elevated temperatures. This technology is similar to solid oxide fuel cells (SOFCs) but operates in reverse to generate hydrogen instead of electricity.
In solid oxide electrolysis, the cell consists of three main components: an electrolyte, an anode, and a cathode. The electrolyte is a solid oxide material, such as yttria-stabilized zirconia (YSZ) or ceria-based materials, which conducts oxygen ions (O2-) at high temperatures (typically above 700 degrees Celsius).
The anode and cathode are porous electrodes that facilitate electrochemical reactions. The anode is usually made of nickel-based materials, while the cathode can be composed of perovskite-type oxides or other mixed ionic-electronic conducting materials.
- Cathode Reaction (Steam Reduction, H2 Production)
- H2O+2e−→H2+O2−
- H2O+2e−→H2+O2−
- Anode Reaction (Oxygen Evolution):
- 2O2−→O2+4e−
- 2O2−→O2+4e−
- Overall, the solid oxide hydrogen electrolysis process can be summarized by the balanced equation:
- 2H2O(steam)→2H2+O2
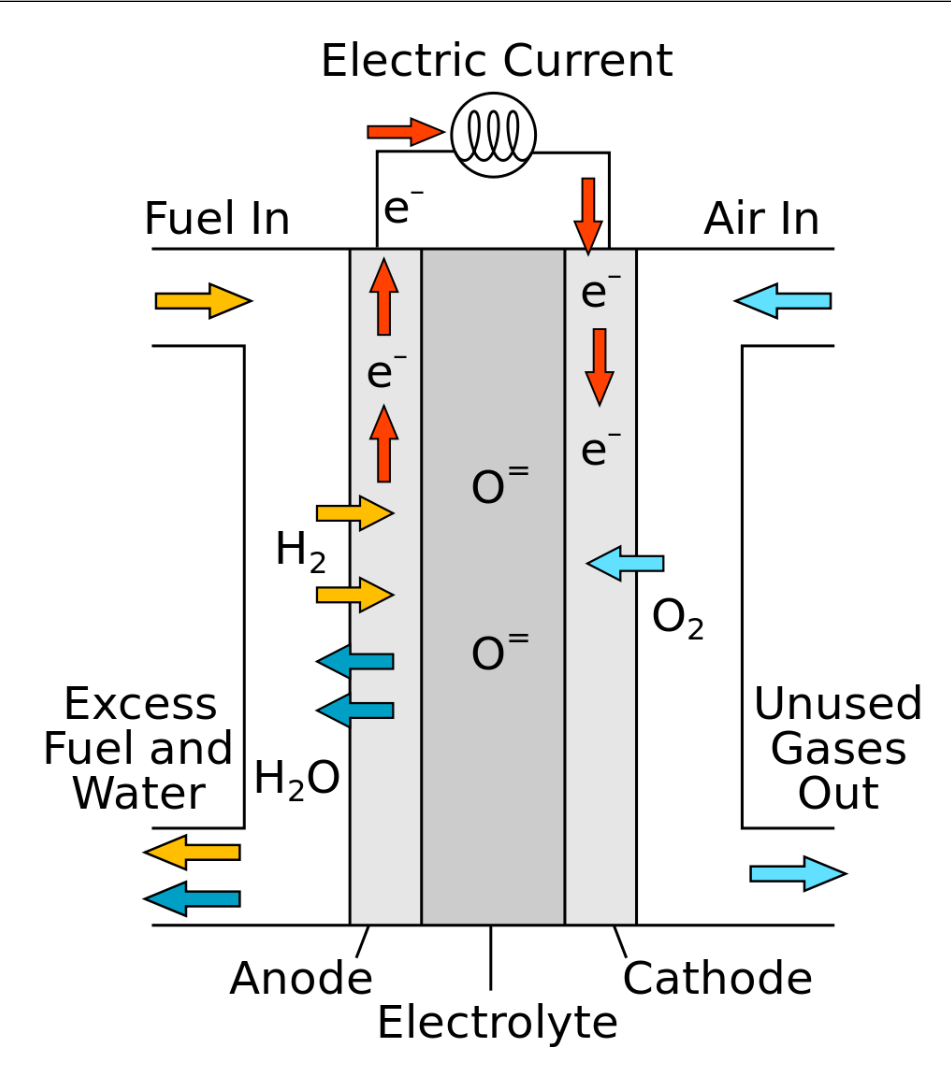
Solid oxide electrolysis operates at high temperatures, which enables faster ion transport through the solid electrolyte, enhancing the overall efficiency of the process. Additionally, the high operating temperatures allow for the utilization of waste heat from industrial processes or other sources, increasing the overall energy efficiency.
The Advantages of Alkaline Electrolysis
Among the various hydrogen electrolysis methods, alkaline electrolysis stands out as a preferred choice for many industries. Here are some reasons why alkaline electrolysis is favored, particularly for industries like glass and ceramics, concrete production, steelmaking, and fertilizers:
- Efficiency: Alkaline electrolysis is known for its high efficiency, making it an economically viable option for large-scale industrial applications. The process converts a significant portion of the electrical energy into hydrogen gas.
- Durability: Alkaline electrolysers have a proven track record of durability and can operate continuously for extended periods. This is crucial for industries with continuous production processes.
- Mature Technology: Alkaline hydrogen electrolysis has been in use for decades and is a mature technology. This means that companies can rely on established systems with a history of successful performance.
- Economic Viability: The relatively low cost of alkaline electrolysers, especially when produced at scale, makes them an economically attractive choice for industrial applications.
- Flexibility: Alkaline hydrogen electrolysis systems are adaptable and can be integrated into existing industrial processes, minimizing the need for major infrastructure changes.
Ceramics-Based Alkaline Electrolysers: A Promising Development
Our scientists and engineers continuously push the boundaries of hydrogen electrolysis technology with groundbreaking innovation. One of the exciting recent developments in alkaline hydrogen electrolysis is the use of ceramics-based electrolysers.
These electrolysers leverage advanced ceramic materials, similar to SOEC but in an alkaline environment, offering several advantages that are encountered in both electrolysis methods:
- High Efficiencies: The use of ceramics allows for efficient ionic conduction, resulting in increased overall hydrogen electrolysis efficiency. This means the same amount of electricity produces more hydrogen.
- Precious Metal-Free: Unlike traditional electrolysers, ceramics-based electrolysers can operate without precious metals like platinum or palladium, reducing costs and reliance on scarce resources.
- Longevity: The durability and resistance to corrosion of ceramic materials make these electrolysers ideal for demanding industrial environments.
Although alkaline hydrogen electrolysis technology is often described as being free of precious metals, the fact is that a large fraction of alkaline electrolysers is still utilising electrodes made of ruthenium and iridium—some of the rarest elements in Earth’s crust.
While such electrolysers boast high current densities and high efficiency, their wider adoption is hindered by raw material availability issues. Nickel-based alkaline electrolysers do not face raw material availability issues but suffer from lower current densities and lower efficiencies. At Stargate Hydrogen, we build upon the best aspects of proven alkaline technology and complement it with breakthrough materials. This results in a completely new class: ceramics-based alkaline electrolysers, which have high current densities, and high efficiencies without precious metals.
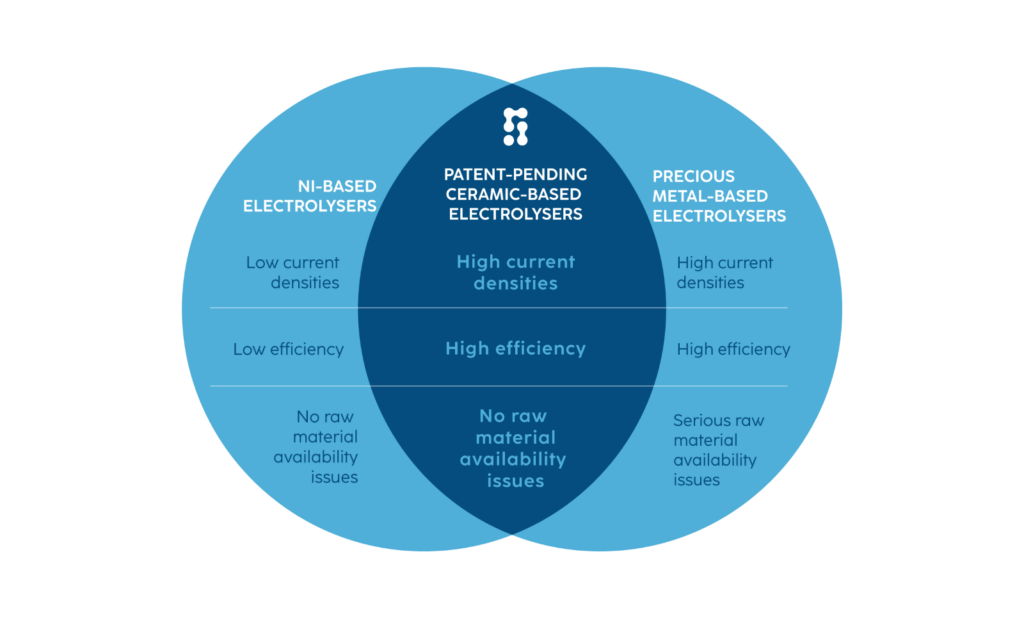
This results in significantly lower hydrogen production costs and makes the electrolysers affordable for the end users. The technology has been validated by several institutions including the Fraunhofer Society.
The Role of Alkaline Electrolysis in Green Hydrogen Production
Green hydrogen production, which involves generating hydrogen using renewable energy sources, is a key component of reducing the environmental impact of various industries. The importance of green hydrogen in modern industrial applications cannot be overstated. Here are some key reasons why green hydrogen is gaining prominence:
- Reduced Carbon Emissions: Green hydrogen production can significantly reduce carbon emissions. When renewable electricity sources like wind or solar power the electrolysis process, the resulting hydrogen is considered "green" and produces no direct carbon emissions.
- Energy Storage: Hydrogen can serve as an energy storage medium, helping to balance energy supply and demand in industries that rely on intermittent renewable energy sources.
- Decarbonising Industrial Processes: Green hydrogen can be used as a feedstock or fuel in industrial processes, reducing the carbon footprint of industries like steelmaking, concrete production, and fertilizer production.
- Clean Transportation: Green hydrogen can be used as a clean fuel for vehicles, especially in sectors where electrification is challenging, such as heavy-duty transport and long-distance travel.
Key takeaways
As the industrial landscape evolves to meet the demands of sustainability and environmental responsibility, hydrogen electrolysis, particularly the alkaline electrolysis method, is poised to play a pivotal role. Companies in industries like glass and ceramics, concrete production, steelmaking, and fertilizers are increasingly recognizing the importance of green hydrogen production.
Green hydrogen offers a pathway to decarbonize industrial processes and is a versatile energy carrier that can be integrated into various applications. Alkaline electrolysis, with its efficiency, durability, and economic viability, is well-positioned to be the technology of choice for these industries.
Our development of ceramics-based alkaline electrolysers represents a significant leap forward in the field. These advanced electrolysers offer the potential to increase productivity while minimising environmental impact.
Hydrogen electrolysis is a vital technology with the potential to revolutionize industries, making them more sustainable and environmentally friendly. Follow our publications to keep up to date on the latest developments in hydrogen production.
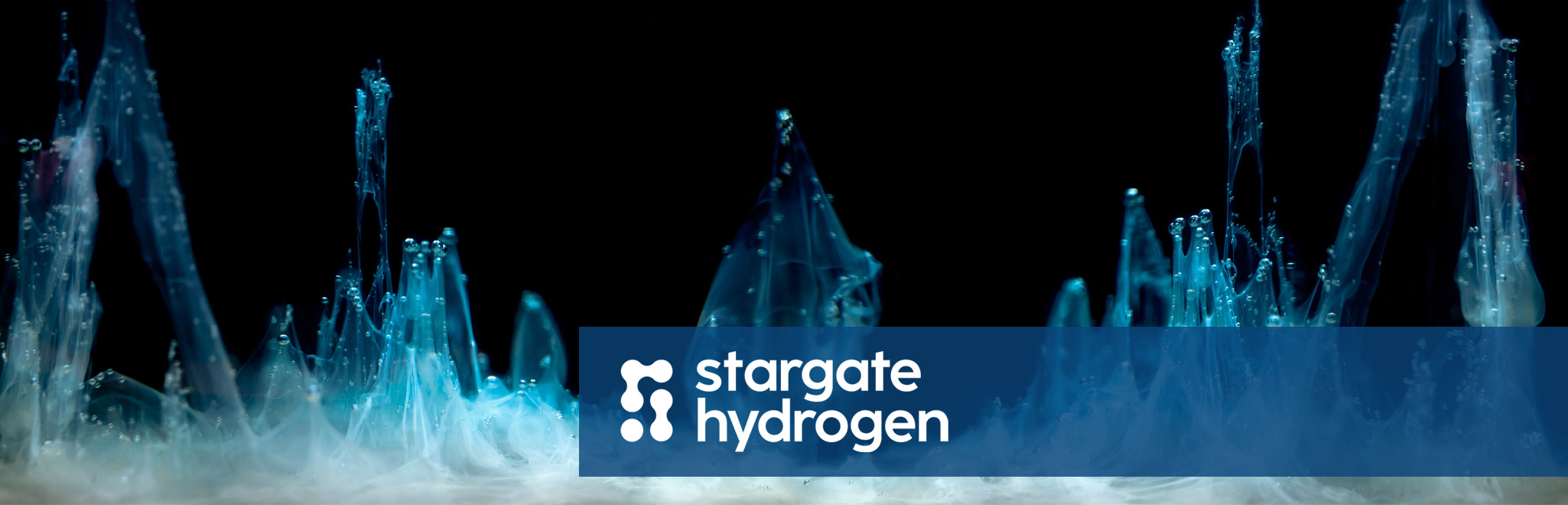